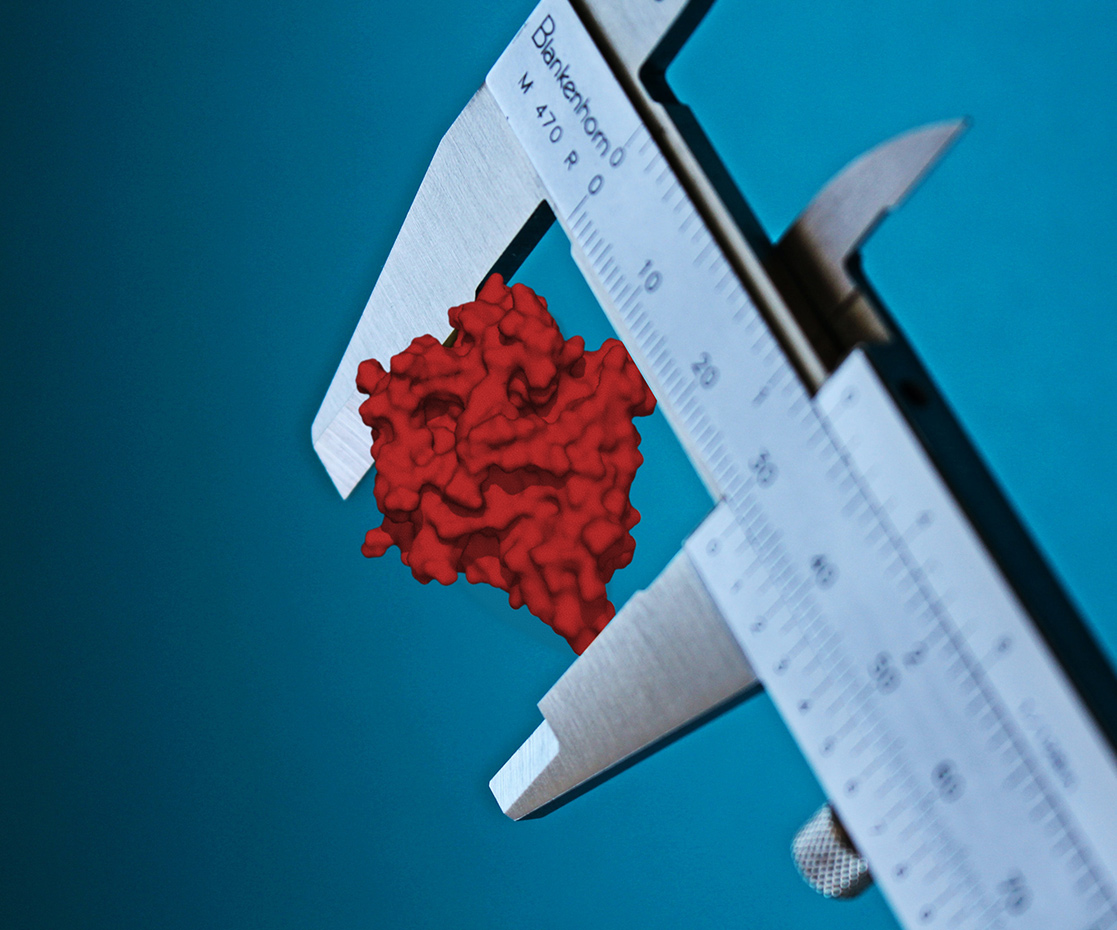
Spin Class: EPR’s Long Distance Relationships
May 16, 2023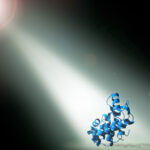
Spin Class: DEER in the Spotlight
July 19, 2023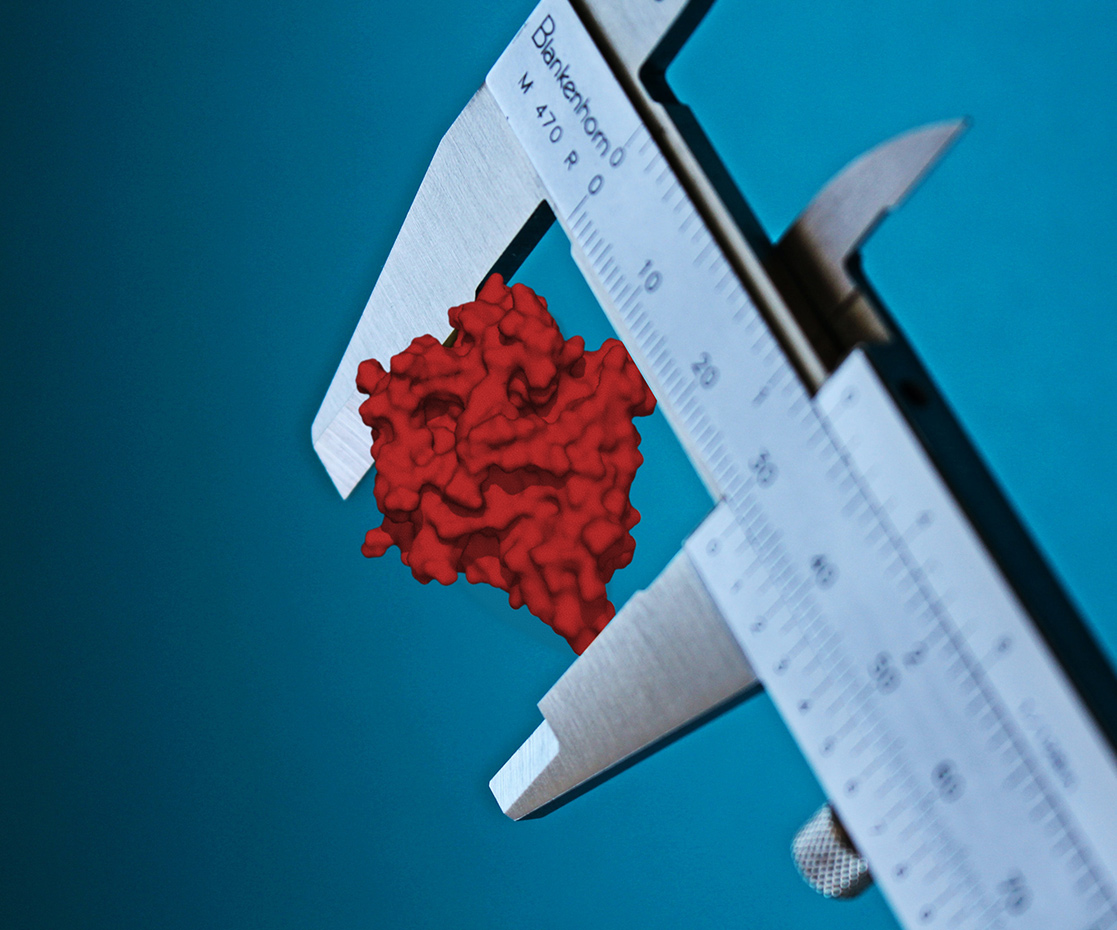
Spin Class: EPR’s Long Distance Relationships
May 16, 2023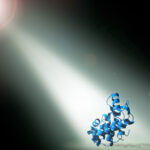
Spin Class: DEER in the Spotlight
July 19, 2023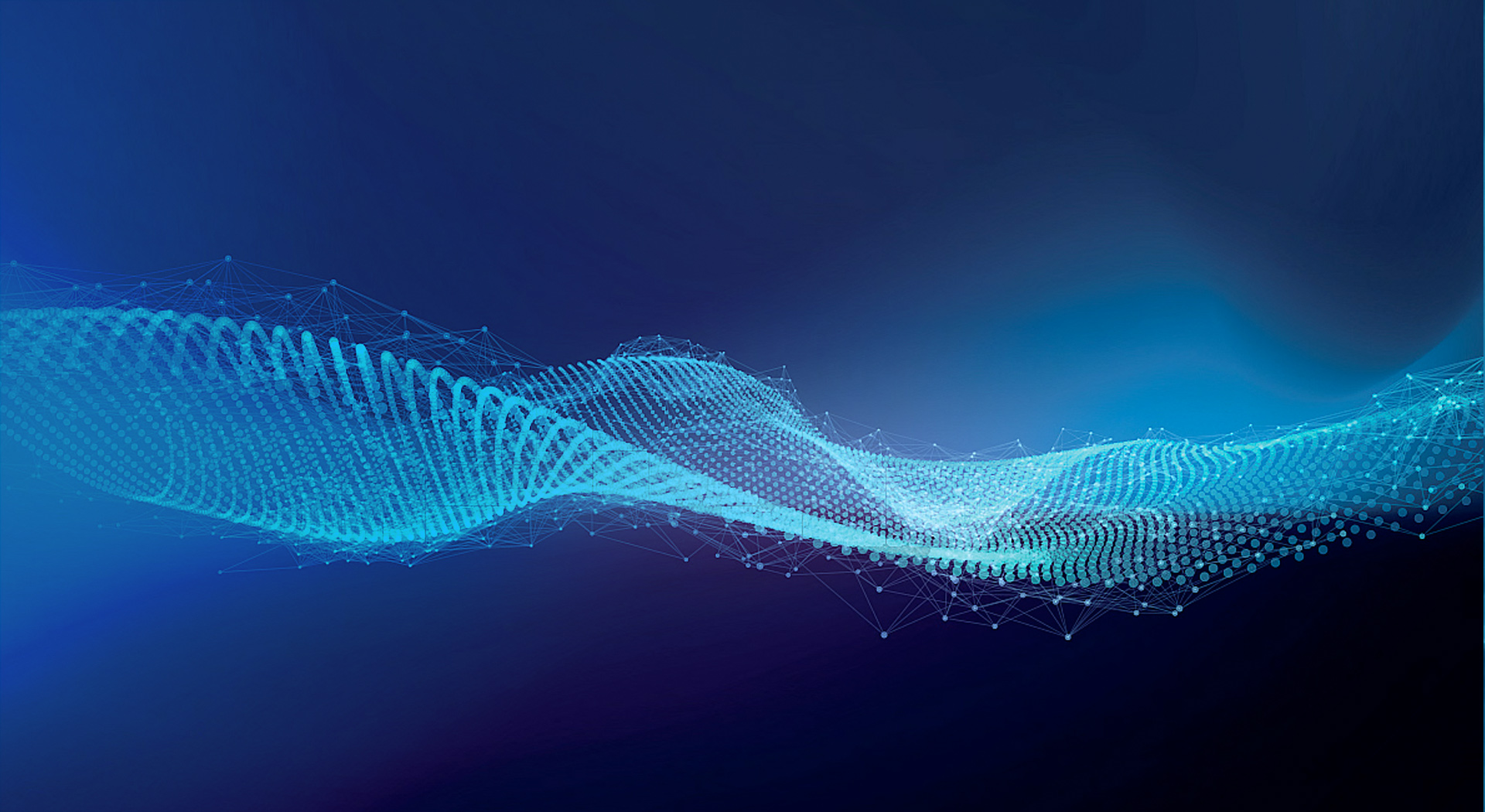
Insights from Austin
The latest insights on EPR technology trends, customer successes and industry best practices
Spin Class: Putting a Label on It
After last month’s general overview of EPR based distance measurements, the next logical topic is the technique responsible for a boom in modern EPR – site directed spin labeling, or SDSL. Many biomolecules of interest lack inherent paramagnetic sites and are thus natively invisible to EPR. To make such systems EPR-detectable, there have been significant and concentrated efforts to develop means by which to incorporate paramagnetic species, either stable organic radicals or paramagnetic metal ions, site-specifically into proteins and nucleic acids. While spin labeling is a nuanced field, I will be introducing the basics of the technique and the three main classes of labels employed today: nitroxides, metal ions, and trityls.
I’ll begin with the venerable nitroxide, the first widely adopted EPR spin label, initially pioneered by Wayne Hubbell of UCLA. In its initial incarnation, cysteine residues were strategically mutated into a protein at specific sites. A nitroxide label containing a methanethiosulfonate group, MTSSL, could then be added to the protein to react with the free thiol groups of the cysteine residues. This reaction results in a disulfide bond that links the paramagnetic nitroxide group to the protein backbone, a sidechain called R1. R1 is responsible for many seminal works in modern EPR and kicked off the field of SDSL development. While R1 is still commonly used, many more nitroxide labels have been implemented, each filling a niche or overcoming a challenge. Attachment to the protein has been varied, using different functional groups and different linkers to the protein to optimize sidechain rigidity or prevent disulfide cleavage (a problem for the rapidly developing field of in-cell EPR measurements). In some cases, cysteine may be foregone for tyrosine – often in cases where the protein has many or functional cysteines – as well as noncanonical amino acids outside of the 21 proteinogenic residues. Use of such alternative foundations requires customized spin labels with functional groups reactive to the amino acid in question. In general, nitroxides are simple, robust, and efficient labels for EPR, with a wide degree of tailorable options to tackle many biophysical problems.
Another major class of labels are paramagnetic metal ions. While many metal ions are EPR detectable, such as Co(II), Fe(III), and Mn(II), the predominant ions used are Cu(II) and Gd(III). Cu(II) is a promiscuous ion, and therefore there are many options for incorporating it into a protein. Chelating tags that coordinate a Cu(II) and then bind to a protein similar to nitroxide labels have been used, but currently Cu(II) labeling can also be achieved through the site-specific placement of two histidine residues, such that Cu(II) will bind specifically to them. This method of an engineered metal binding site is advantageous in its bidentate approach, which constrains the mobility of the Cu(II) label and produces EPR distance measurements much narrower than most other labels. The inherent rigidity of this scheme provides information directly on the protein backbone and is not convoluted behind the flexibility of the label. The other predominant metal ion, Gd(III), exhibits a narrow central peak in its EPR spectrum which provides enhanced sensitivity in distance measurements over broader species like Cu(II), while also being relatively stable in-cell, making it an attractive label for physiological EPR. Gd(III) is typically incorporated into the protein of interest via a bifunctional label, with one end containing a Gd(III) chelating group, and the other containing a reactive group to form the protein-label linkage. As mentioned earlier, this linkage may vary, as disulfide bonds are prone to being cleaved in the cellular environment and thus cleavage-resistant linkers are often employed.
More recently, another organic radical has gained significant attention for SDSL – the trityl, or triarylmethyl radical. Trityls have the general structure of a central carbon radical with three spokes consisting of aromatic rings. Trityls are especially notable for their properties with regards to in-cell EPR and room temperature measurements. In-cell measurements are important to determine structural insights within the native, crowded environment of the cell. This same environment is harsh and often reducing, turning radicals into their EPR-silent analogs. Trityls, being larger and more sterically protected than typical nitroxide labels, have more favorable redox properties, and can exhibit long lifespans within the cell. Likewise, they have very narrow EPR spectra which makes measurements on trityls much more sensitive than on broader species, analogous to Gd(III). Finally, trityls also possess excellent relaxation characteristics, which makes their EPR signal detectable at room temperature, whereas most other labels require cryogenic temperatures for EPR experimentation.
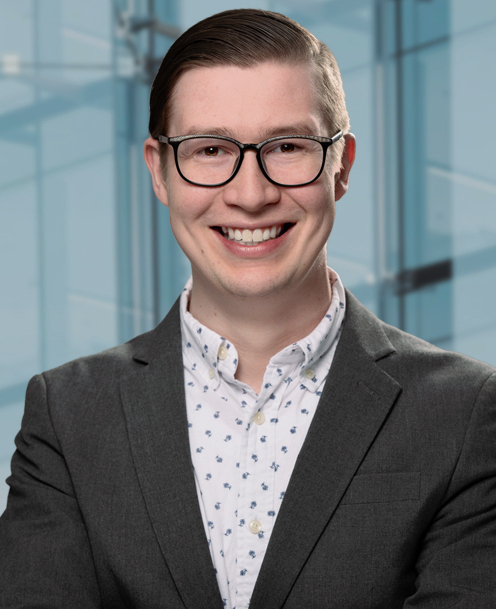
Austin Gamble Jarvi
Applications Scientist
There is much more to be said on this topic given the exhaustive information on protein labeling, optimizations, orthogonal labeling strategies, photoactivatable labels, and more. This is not to mention the tremendous efforts of labeling nucleic acids, lipids, and other molecules. However, I hope this post helps you to further dip your toe into the EPR pool or serves as a gateway to further investigation. The inception of SDSL incited a rapid expansion within EPR, enabling its application to systems unreachable before. With each spin label type bringing unique advantages and applications, EPR spectroscopists possess a broad arsenal to investigate protein structures, dynamics, and interactions. Till next time!